Collaborative Research Shows Good Bacteria Can Be Protected in Fight Against Infections
Interdisciplinary team found how changing the shell of bacterial organelles can influence their function
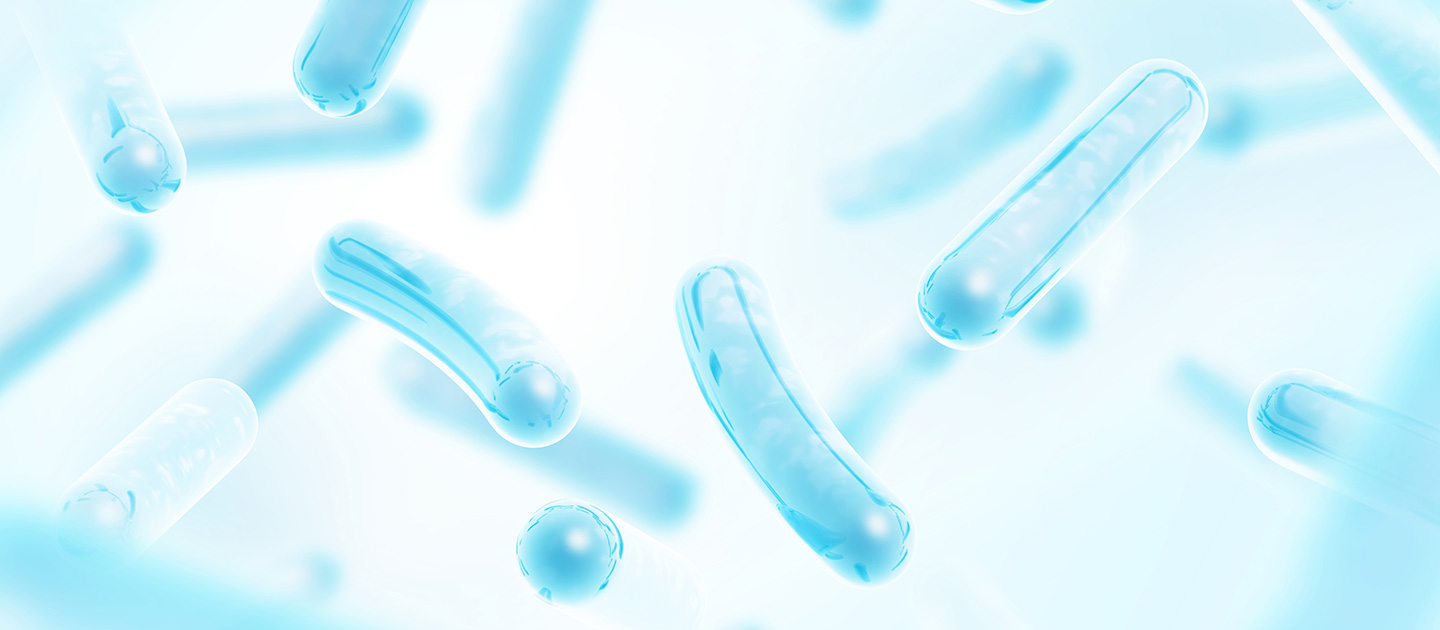
One of the many challenges of medical science is creating antibiotics that kill infectious diseases but leave useful bacteria alone. Even if a treatment is effective at neutralizing the intruder, its benefits are neutralized if it also attacks healthy cells.
Recent work from an interdisciplinary team of Northwestern Engineering researchers showed that the geometry and function of a bacterial organelle can be altered to inhibit the growth of harmful bacteria involved in illnesses.
Led by the McCormick School of Engineering’s Danielle Tullman-Ercek, the investigators studied the components that make up the shell of bacterial organelles called microcompartments. They found how the geometry of the resulting structures can be altered with small changes to the components, as well as how that geometry change influences their function.
“Given that 12 of the 14 pathogens that cause the most threatening infectious diseases have these microcompartments, compared to only around 30 percent of bacteria more generally, the systems are hypothesized to be a potential antibiotic target that would allow pathogens, but not all the ‘good’ bacteria, to be killed,” said Tullman-Ercek, professor of chemical and biological engineering. “Our work indicates these systems are promising antibiotic targets and, from an engineering biology standpoint, that we now understand more rules for how to alter the geometry to make nanostructures of all shapes and sizes.”
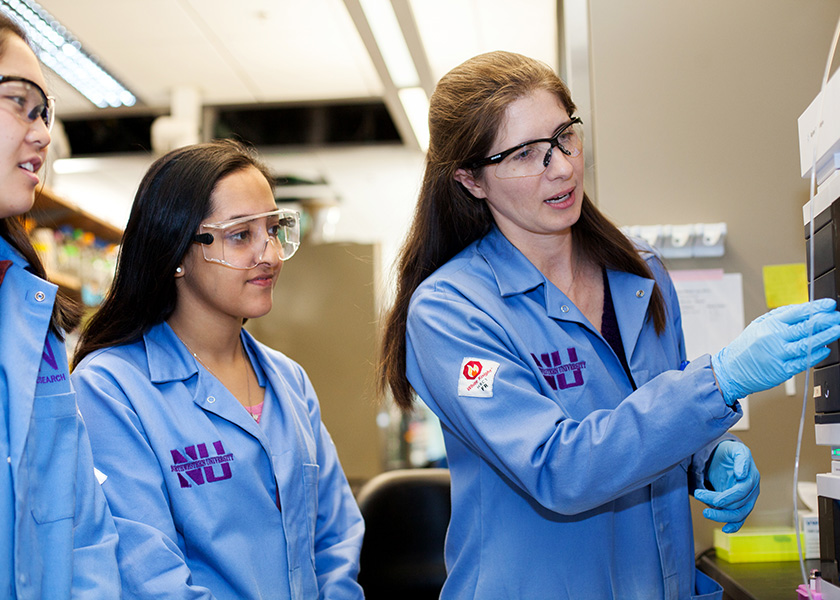
The research was released in two papers. One study, “Linking the Salmonella Enterica 1,2-propanediol Utilization Bacterial Microcompartment Shell to the Enzymatic Core via the Shell Protein PduB” was published May 16 in Journal of Bacteriology and was co-authored by Tullman-Ercek along with Michael Jewett, Walter P. Murphy Professor of Chemical and Biological Engineering, and Niall Mangan, assistant professor of engineering sciences and applied mathematics.
The other study, titled “Vertex Protein PduN Tunes Encapsulated Pathway Performance by Dictating Bacterial Metabolosome Morphology,” was published June 29 in Nature Communications. The paper was co-authored by Tullman-Ercek, Jewett, Mangan, and Monica Olvera de la Cruz, Lawyer Taylor Professor of Materials Science and Engineering and Chemistry. That piece was included in an Editors’ Highlights webpage of recent research called “Biotechnology and methods" on the Nature Communications website.
Understanding the rules of virus self-assembly
The work builds off previous research by Tullman-Ercek and her collaborators.
In 2019, Tullman-Ercek expanded the understanding of how virus shells self-assemble, an important step toward developing techniques that use viruses as vehicles to deliver targeted drugs and therapeutics throughout the body. By performing multiple amino acid substitutions, the researchers discovered instances of epistasis, a phenomenon in which two changes produce a behavior different from the behavior that each change causes individually.
Last year, researchers theoretically and experimentally investigated protein assembly to build microcompartments of different shapes and sizes, including long tubes and polyhedra. That work illuminated how biological units composed of proteins, such as viruses and bacterial organelles, develop. It also informed new ways to design medicine, synthetic cells, and nanoreactors.
Those investigations set the stage for this collaborative effort to help good bacteria survive.
“Prior to these two studies, we worked closely with the Olvera de la Cruz and Mangan groups to understand how bacterial organelles function,” Tullman-Ercek said. “In previous work, their computational modeling expertise helped us generate hypotheses which we tested experimentally, both about how bacterial organelles are built in cells as well as how they carry out their important cellular functions. In these most recent studies, their input helped us understand experimental findings that two genes are responsible for different aspects of bacterial organelle formation and function.”
Structural proteins dictate organelle performance
In “Linking the Salmonella Enterica 1,2-propanediol Utilization Bacterial
Microcompartment Shell to the Enzymatic Core via the Shell Protein PduB,” researchers found that PduB, an important gene, encodes a protein that links the outer shell of the organelle (think of an eggshell) to the inner, functional components of the organelle. In the absence of this protein, empty organelles are formed in cells completely devoid of their functional innards.
The Mangan group leverages mathematical modeling of biologically driven chemical reactions to understand how spatial organization in cells can impact their survival and proliferation. To accurately evaluate the bacteria’s behavior, Tullman-Ercek turned to Mangan’s group, which already had models of the kinetic reactions that happen in bacterial compartments.
“During our experiments we noticed an interesting trend; certain mutant strains of bacteria had unexpected growth trends,” said Carolyn Mills, a postdoctoral researcher in Tullman-Ercek’s lab who was the first author on the PduN study. “Niall’s expertise in computationally modeling these growth trends helped point us in a direction we hadn’t previously considered and wound up being correct. This saved us months of time doing experiments.”
Two members of Mangan’s group, graduate student Andre Archer and postdoc Sasha Shirman, developed dynamic models of the metabolic pathway of interest in Tullman-Ercek’s experimental system. These models can predict the concentration of different metabolites over time for different reaction rates and spatial organizations. They were used to help identify whether changes in the spatial organization (inside compartments vs. outside) or changes in enzyme concentration led to differences in metabolite concentrations and growth of the bacteria.
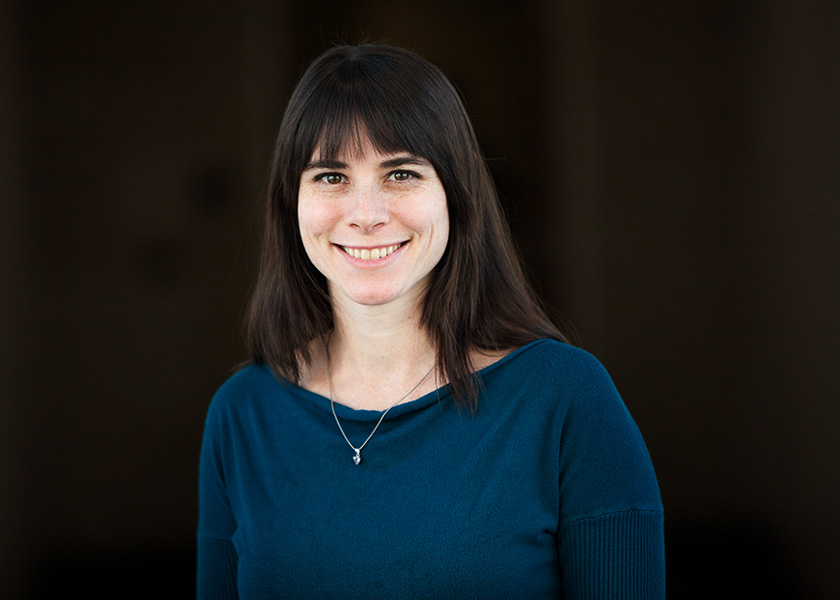
“While the model can't tell you which is happening in a cell, it can tell which changes in systems parameters can lead to the observed behavior,” Mangan said. “In this case we identified that a change in enzyme concentration could lead to the observed behavior – a hypothesis that had not been previously considered. Danielle’s group was then able to test experimentally and show that enzyme concentration had changed and therefore, was likely the main source of the unexpected behavior.”
Tullman-Ercek and Mangan have collaborated for six years. That interdisciplinary work, Mangan said, has significant value.
“I like to work on interdisciplinary teams because each group has different skill sets and it allows us to ask and answer questions that we couldn't address independently,” Mangan said. “I really enjoy the process of talking with others about their systems and experiments and trying to figure out if there is a way to use modeling to ask new questions or provide our collaborators with insight that they couldn't get otherwise.”
Decoding how molecular interactions lead to structural changes
In “Vertex Protein PduN Tunes Encapsulated Pathway Performance by Dictating Bacterial Metabolosome Morphology,” the researchers found that a structural protein, PduN, encoded by a gene is responsible for imparting the shape of these organelles – in the absence of this protein, the organelles form highly-elongated structures, skewering cells like shish kabobs.
The Olvera de la Cruz group has expertise in simulating molecular-scale physical processes. These simulations allowed Tullman-Ercek’s team to understand and characterize interactions that are inaccessible experimentally. In this study, the Olvera de la Cruz group used these types of simulations to provide molecular-level explanations of why the shapes of bacterial organelles changed upon loss of one key protein.
“The insights we gained from this work continue to help us understand the complex combination of factors that impact how bacterial organelles are built in cells,” Tullman-Ercek said. “They proposed mutations we could make. Instead of getting rid of the entire protein, we could prove it was really that interaction that mattered by changing one amino acid at the interface. That was important, and so we made the changes and they modeled those changes. It was a back and forth. It was truly collaborative work.”
Next steps
The work, however, isn’t complete.
Tulllman-Ercek’s group is working with Olvera de la Cruz’s lab to model the full process of organelle formation. That project, Tullman-Ercek said, has benefited immensely from working together closely and experimentally testing hypotheses generated from simulations.
In the future, we envision modifying these organelles to create designer probiotics capable of treating human diseases. Professor of Chemical and Biological Engineering
With the Mangan group, Tullman-Ercek’s group continues to explore new chemical reactions for sustainable production and how those benefit from spatial organization in organelles. In the future, they envision that coupling understanding of bacterial organelle formation with chemical reaction modeling will enable rapid optimization of bacteria for sustainable bioproduction.
These developments will move the researchers closer to repurposing bacterial organelles.
“These are useful modules that can help bacteria realize their potential as sustainable cellular factories. By understanding the essential components of these organelles, how they come together, and how they can be modified, we can redesign them to fit specific purposes,” Tullman-Ercek said. “In the future, we envision modifying these organelles to create designer probiotics capable of treating human diseases.”