Scientists Uncover Major Cause of Resistance in Solid Electrolytes
Findings could lead to new methods to design more efficient batteries and fuel cells
Northwestern Engineering and Argonne National Laboratory researchers have uncovered the underlying causes of the detrimental resistance of grain boundaries in a solid electrolyte material. The study involved two powerful techniques — electron holography and atom probe tomography — that allowed scientists to observe the boundaries at an unprecedentedly small scale. The resulting insights provide new avenues for tuning chemical properties in the material to improve performance.
Most solid electrolyte materials consist of hundreds of thousands of small crystalline regions, called grains (much like crystalline grains of salt), each with a different orientation. The materials, used in fuel cells and batteries, conduct electricity by transporting ions, or charged atoms, from one side of the electrolyte to the other. Boundaries between the grains in the materials are known to impede the flow of ions through the electrolyte, but the fundamental reasons for this resistance have remained hidden.
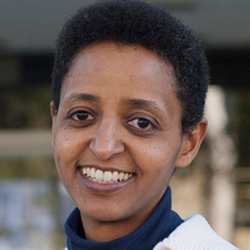
A study describing the work, titled “Variability and Origins of Grain Boundary Electric Potential Detected by Electron Holography and Atom Probe Tomography,” was published on April 13 in Nature Materials. Xin Xu (PhD ’19), a former graduate student in Haile’s group, was the study’s first author. Northwestern Engineering’s Vinayak Dravid, Abraham Harris Professor of Materials Science and Engineering, also contributed to the research.
“When scientists study the conductivity of these electrolytes, they typically measure the average performance of all of the grains and grain boundaries together,” said Charudatta Phatak, a scientist in Argonne’s Materials Science Division (MSD) and coauthor on the study. “But strategically manipulating the material properties requires deep knowledge of the origins of the resistance at the level of individual grain boundaries.”
To explore the grain boundaries, the scientists performed electron holography of a common solid electrolyte at Argonne’s Center for Nanoscale Materials (CNM), a US Department of Energy (DOE) Office of Science User Facility. In this process, a beam of electrons hits a thin sample of the material and experiences a phase shift due to the presence of a local electric field in and around it. An external electric field then causes a portion of the electrons passing through the sample to be deflected, creating an interference pattern.
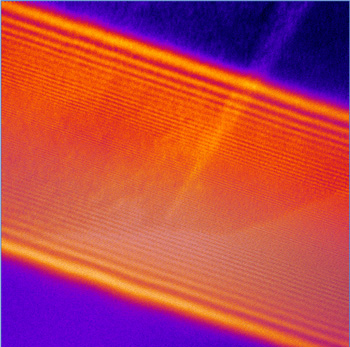
Before this study, scientists thought that resistance at grain boundaries arose due to internal thermodynamic effects alone, such as the limit on the build-up of charge in an area. However, the large and varied electric fields they observed indicated the existence of previously undetected impurities in the material that explain the resistance.
“If the resistance was only due to thermodynamic limits, we should have seen the same fields across different boundary types,” Phatak said. “Since we saw differences of almost an order of magnitude, there had to be another explanation.”
To further study the trace impurities, the scientists used the Northwestern University Center for Atom Probe Tomography (NUCAPT) to determine the chemical identity of individual atoms at the grain boundaries. The electrolyte material in the study, made of ceria and often used in solid oxide fuel cells, was thought to be almost completely pure, but the tomography revealed the existence of impurities including silicon and aluminum — produced during material synthesis.
“On the one hand, it shows that if you make your materials cleaner, you can lessen these interfacial problems with electrolytes,” Haile said. “Realistically though, you can’t make a sample at an industrial scale cleaner than what we had prepared.”
These inherent impurities are configured at the grain boundaries in a way that causes the electric fields across the boundaries to resist the flow of ions. The footprints that the impurities leave on the overall resistance of the electrolyte closely resemble what scientists would expect from thermodynamic effects alone. Understanding the true cause of the resistance — the impurities — can help the scientists to correct for it.
“Based on our findings, we can intentionally insert elements into the material that negate the effects of the impurities, lowering the resistance at the grain boundaries,” Phatak said.
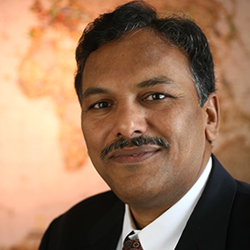
“If ions can move across the interfaces of these solid-state electrolytes more effectively, batteries will become much more efficient,” said Haile, codirector of the Institute of Sustainable Energy at Northwestern (ISEN). “The same is true of fuel cells, which is closer to the material system we studied. There’s a potential to really impact fuel efficiency by making it easier to operate at temperatures that aren’t extremely high.”
Funding for the study, in part, came from a Northwestern-Argonne Early Career Investigator Award for Energy Research awarded to Phatak. The program, which was matched by funds from ISEN, fostered a collaboration between Phatak and Haile and supported Xu’s contributions.
The Division of Materials Science and Engineering (DMSE) of the DOE Office of Science’s Basic Energy Sciences (BES) program also supported Phatak’s work on the project. Additional support was provided by Northwestern’s NSF-funded Materials Research Science and Engineering Center as a seed award to Haile upon her transition to Northwestern from another institution.