Reprogramming the Genome
New Center for Physical Genomics and Engineering ignites a revolution in medical research and beyond.
“I hope within years, our technologies can make a significant difference for a generation of patients, including cancer patients.”
Imagine taking a DNA molecule — the source of genetic instructions for all living things — and making it a million times larger. The result would be about the width of a spaghetti noodle, but the length would travel from New York City to Dallas.
Now imagine taking that long DNA noodle and stuffing it into a small living room. That illustrates the highly-packed, dense structure of what is called chromatin, the three-dimensional organization of the genome which regulates patterns of gene expression.
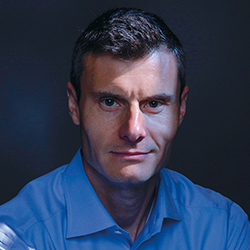
Their groundbreaking work is advancing an entirely new field called physical genomics. The findings to date have been striking: when researchers targeted chromatin structure to limit a cancer cell’s ability to evolve and become resistant to chemotherapy drugs, the technique eliminated virtually 100 percent of cancer cells in cell cultures and animal models.
Armed with these initial results and with an eye toward tackling some of today’s most complex medical problems, Backman is launching the Center for Physical Genomics and Engineering, a new collaborative force that will further secure Northwestern’s spot at the forefront of this emerging field. “I hope within years our technologies can make a significant difference for a generation of patients, including cancer patients,” says Backman, Walter Dill Scott Professor of Biomedical Engineering at the McCormick School of Engineering.
Hacking the Body’s Software for Good
Researchers have long known that the root of many of the intractable diseases that afflict human beings lies within their genes. In recent years, gene editing tools like CRISPR and Cas9 have been developed to try to solve this problem.
With these tools, researchers have asked: What if biomedical engineers could find a way just to edit out the genes that are associated with incurable or debilitating diseases? What if researchers in the field of epigenetics could simply turn on the genes they want expressed, and turn off the ones they don’t?
“Physical genomics falls right at the interface of physical sciences, biology, and computational sciences. You can’t address it within the context of any one existing field of science. You need a convergence. Collaboration is critical. Nobody can do it alone.”
Even if researchers could achieve all that, they still would have solved only part of the problem because they wouldn’t have taken an overall systems view: Many diseases involve an interplay among hundreds or even thousands of genes, all influenced by the structural organization of chromatin. Physical genomics aims to do just that — to take an all-encompassing approach and regulate global patterns of gene expression.
If we consider genes to be the body’s hardware, then chromatin is its software, and the structure of chromatin is the operating system. Only by understanding how all three work together can researchers hope to take on complex diseases like cancer, which can involve the entire genome.
For the past 20 years, Backman has tried to understand just how this genomic software works. “To start addressing these questions, we had to develop the technologies first, which took a long time,” he says. Those technologies include nanoscale super-resolution imaging, computational modeling of complex biological processes, and computational electrodynamics, all developed at Northwestern by researchers from disparate disciplines working together.
“Physical genomics falls right at the interface of physical sciences, biology, and computational sciences,” Backman says. “You can’t address it within the context of any one existing field of science. You need a convergence. Collaboration is critical. Nobody can do it alone.”
To develop the needed technologies, Backman teamed up with researchers across Northwestern, including Allen Taflove, professor of electrical and computer engineering. For more than 40 years, Taflove has been solving Maxwell’s equations, which are fundamental equations of classical electrodynamics.
Taflove and Backman have collaborated over the past 15 years, and Taflove helped with initial work using light to detect nanoscale changes in tissue that indicate cancer. Taflove is now working to use that technology to detect changes in chromatin.
When the technologies were refined to the point that researchers could begin looking at never-before-seen parts of the genome, Backman and his collaborators began modeling the processes in play there, and a whole new world of possibilities opened up. “The research has shifted in an extremely exciting way,” Taflove says. “How often does an engineer have the opportunity to say that we can really change the world? This center could spark a revolution in medicine.”
Developing a New Cancer Therapy
The team’s first target is cancer. Through the nanoimaging technologies, researchers discovered that the packing density of chromatin in cancer produced predictable changes in gene expression. In other words, the more disordered the density, the more likely the cancer cells were to adapt and survive, even in the face of chemotherapy. If their packing density was more ordered, the cancer cells were more likely to die from treatment.
Northwestern researchers realized they could control the chromatin by changing the electrolytes in the cell’s nucleus using FDA approved drugs already on the market. The drugs were prescribed for arthritis and heart conditions, but they also had a side effect of altering chromatin packing density.
Researchers call the technique chromatin protection therapy and found when they used it in conjunction with chemotherapy drugs in both cell cultures and animal models, it almost completely eliminated cancer cells. The combination regulates chromatin’s structure in a way that prevents cancer from evolving to withstand treatment, making the disease an easier target for existing drugs. “In the near term, this could become a broad spectrum therapy for cancer,” Taflove says.
Harnessing the Power of the Genome
Cancer isn’t the only target for this type of therapy. Other complex diseases, like Alzheimer’s and atherosclerosis, could potentially be treated by regulating global patterns of gene expression.
In fact, given that every organism is ultimately defined by its genome, the technique could be used in any living thing for a variety of purposes. Backman speculates that perhaps plants could be altered to better withstand climate change, or cells could be altered to create new kinds of fuels.
One of most encouraging aspects of physical genomics is that, unlike gene editing, the results are completely reversible. If genes are the hardware, then editing any gene would make the changes permanent. But, Backman adds, if chromatin is the software, and chromatin structure is the operating system, then changes can be dynamically altered and shifted, then changed back, even in real time.
For collaborator Guillermo Ameer, Daniel Hale Williams Professor of Biomedical Engineering and Surgery and director of the Center for Advanced Regenerative Engineering, the technology could help him and his team better understand how cells interact with surfaces and each other, leading them to design better materials to promote regeneration in the body.
“This is a new frontier,” Ameer says. “We have the opportunity at Northwestern to be at the center of what could impact millions down the road.”
For researchers in the Feinberg School of Medicine’s Department of Obstetrics and Gynecology, the center could further their goal of successfully treating ovarian cancer, a disease that is particularly difficult to cure. Backman has worked with Daniela Matei, Diana, Princess of Wales Professor of Cancer Research, to use his technology to image the chromatin of ovarian cancer stem cells to see how the chromatin structure changed with treatment. Backman is also working with Shohreh Shahabi, John and Ruth Brewer Professor of Gynecology and Cancer Research, to develop a clinical trial to use his chromatin protection therapy in ovarian cancer patients.
“Vadim goes out of his way to make collaborations possible,” Matei says. “He opened the door for us to explore this new therapy, and the new center will only facilitate more of these intersections between engineering and translational oncology.”
Because the nature of physical genomics research is so interdisciplinary, one of the goals of the new center is to educate the next generation of researchers who can think and communicate across disciplines. “The traditional educational paradigm is not the best to train highly interdisciplinary researchers,” Backman says. “You need an engineer who can think like a biologist and know enough about biology to talk with biologists, and vice versa.”
The center hopes to develop curricula and training for high school students all the way to postdoctoral fellows that will help develop thinkers who can speak multiple scientific languages. “It’s one of the best times ever to begin studying engineering,” Backman says. “With knowledge of the convergence of all these fields, like artificial intelligence, biotechnology, physical sciences, and medicine, engineers will be the leaders in this new world. There’s no doubt about it.”
Though much work remains to be done, Backman and his collaborators hope that, through the efforts of the Center for Physical Genomics and Engineering, this technology and the therapeutics it provides could be in the hands of physicians for clinical use within a decade.
“My goal is to save one patient,” he says. “And then we’ll go from there.”