Reconfiguring Active Particles into Dynamic Patterns
Electrostatic imbalance causes particles to swarm, cluster, and form connected chains
From swarming bees to clustering bacteria colonies, nature stuns with its ability to self organize and perform collective, dynamic behaviors. Now researchers have found a way to mimic these behaviors in active materials on the microscale — by varying just a single parameter.
Northwestern Engineering’s Erik Luijten and Steve Granick of Korea’s Institute for Basic Science (IBS) and Ulsan National Institute of Science and Technology demonstrated control over the interactions among microscopic spheres — causing them to self-propel into swarms, chains, and clusters. Such active particles could open a new class of technologies with applications in medicine, chemistry, and engineering as well as advance scientists’ fundamental understanding of collective, dynamic behavior in systems.
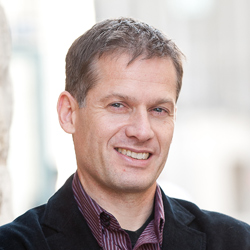
“We are taking small steps toward encouraging lifelike behavior in materials,” said Granick, who directs the IBS Center for Soft and Living Matter. “We are already beginning to see that active materials can behave intelligently.”
Supported by the Korean Institute for Basic Science, the Department of Energy, the National Science Foundation, and Northwestern’s Materials Research Center, the research is described July 11 online in Nature Materials. Ming Han, a PhD student in Luijten’s laboratory, and Jing Yan, a former graduate student at the University of Illinois, served as co-first authors of the paper.
Luijten and Granick both emphasized the importance of team work as this current discovery is part of a longtime collaboration using a new class of soft-matter particles called Janus colloids, which Granick previously developed in his laboratory. Luijten’s team completed the theoretical computer simulations, and Granick used his colloids to experimentally test the collective, dynamic behavior in the laboratory.
“This really was a joint work,” Luijten said. “Without either the experimental or the computational contribution, it would not have succeeded.”
Named after the Roman god with two faces and typically suspended in solution, the micron-sized spheres have attractive interactions on one side and are negatively charged on the other.
“Colloids are a great model system,” Luijten said. “Real materials, such as molecules, are very difficult to see and manipulate. These colloids have similar behaviors but on timescales and length scales that we can access. Even though they are simple, their behavior is representative for systems.”
By subjecting the colloids to an electric field, the electrostatic interactions between the two sides of the self-propelled spheres could be manipulated. Some experienced stronger repulsions between their forward-facing sides. Others experienced the opposite. And yet another set remained somewhere in the middle. This imbalance caused the self-propelled particles to “swim” and self-organize into one of four different patterns: a random gas, swarms, clusters, or chains.
To avoid head-to-head collisions, head-repulsive particles swam side-by-side, aligning into swarms. Tail-repulsive particles positioned their tails apart, jamming their heads together to form clusters. And swimmers with equal-and-opposite charges attracted one another into connected chains.
“These types of behavior have never been seen before in a single system,” Luijten said. “We were able to demonstrate all these different classes of behavior in one system by turning just one knob.”
This discovery could have various applications in drug delivery, sensing, or even microrobotics. A drug could be put inside particles, for example, that cluster into the spot of delivery. Or changes in the environment could be sensed if the system suddenly switches from swarming to forming chains.
“If you want to complete tasks on the micron scale, it’s difficult to insert a chip into a particle or program a particle that small,” said Han. “So it’s necessary to find the simplest way to control all those patterns.”