New Material Combines Useful, Incompatible Properties
Material is based on a theory developed by James Rondinelli
Mild-mannered Dr. Jekyll and malicious Mr. Hyde were opposite aspects of the same man, and their story ended in tragedy because the two couldn’t peacefully coexist.
Most materials, too, are capable of being only one thing at a time, but a team of engineers and physicists, including Northwestern Engineering’s James Rondinelli, has created an entirely new material in which completely contradictory properties can coexist. Chang-Beom Eom, a professor of materials science and engineering at the University of Wisonsin-Madison, led the research.
The compound, which the researchers described in a study published April 20 by the journal Nature, is a polar metal. Although polar metals should not be possible, they do occur in nature.
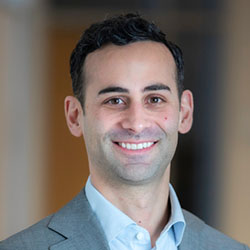
Undeterred by the laws of the universe, the research team created a compound that is a scientific oxymoron. Through a new synthesis approach supported by detailed theory and computational modeling, the group made a crystal with multiple personalities: part polar, part metallic.
Metals conduct electricity because electrons flow freely throughout them. Polar materials, by contrast, frequently impede the free flow of electrons and work as electrical insulators.
The researchers needed to find something that can demonstrate both insulating and conducting properties. First, they separated the polar and metallic parts of the crystal. Some electrons gave rise to the metallic nature, moving within the material to conduct electricity. Other electrons contributed to the polar properties.
However, because the natural molecular structure of the material is symmetrical, even after separating the two components, the material as a whole would not be polar. Equal and opposite arrangements of ions and electrons canceled each other out. To overcome this obstacle, the researchers synthesized the substance with slightly off-kilter atoms, which threw off the internal symmetry enough to make the material polar.
During this process, Rondinelli and his postdoctoral fellow Danilo Puggioni used a set of operational principles for the design of polar metals that they theoretically proposed in 2014. The design approach allowed the team to circumvent fundamental electrostatic principles, which are routinely taught in college level physics courses. These ceramic materials are able to both conduct electricity and exhibit structures without inversion symmetry owing to local dipoles created from an asymmetric arrangement of negative and positive charge — just as a water molecule exhibits a dipole — in the crystal. The contraindication of metallicity and broken inversion symmetry is overcome by decoupling how those dipoles are created from the electrons that provide the electrical conduction. The design concepts provide a new platform from which to realize functional materials with properties that are often challenging to make coexist together.
“It’s exciting to see our theory used in the laboratory with the power of quantum mechanical computational methods to predict and understand the link between atomic-scale structure and properties,” Rondinelli said.
Ultimately, the team made the polar metal by painstakingly growing thin films of material one atom at a time. Crucially, they grew the substance on top of a supporting lattice with a slightly offset molecular organization. Tightly clamping the growing film to this support skewed the internal arrangement of their material, stabilizing its internal geometry in the asymmetrical orientation necessary to maintain polarity.
Synthesizing and characterizing this first-of-its-kind material required patience and precision. The researchers counted every atom deposited on the surface, as the substance slowly grew one layer at a time. They then used multiple complex optical and electronic and structural measurements to determine its properties.
Their approach is an attempt to accelerate the discovery of multifunctional materials with unusual coexisting properties, paving the way to devices with the ability to perform simultaneous electrical, magnetic and optical functions.
This work was supported by the National Science Foundation under the Designed Materials to Revolutionize and Engineer our Future program, the US Department of Energy Office of Basic Energy Sciences, and the Army Research Office. University of Wisconsin physics professor Mark Rzchowski, Venkatraman Gopalan of Penn State, Xiaoqing Pan of University of California at Irvine, Craig Fennie of Cornell University, and Hua Zhou, Phillip Ryan, Yongseong Choi, and Jong-Woo Kim of Argonne National Laboratory also contributed to the study.
— Adapted from a press release by the University of Wisconsin-Madison