More Than the Sum of its Parts
Northwestern scientists and engineers work to understand and predict the behaviors of complex systems
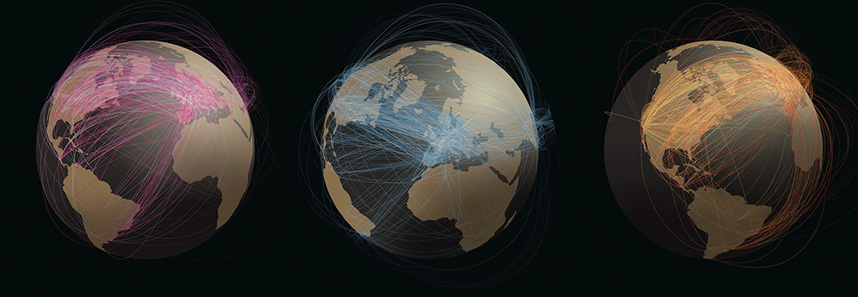
By itself, one neuron is nothing but a star-shaped cell in a dish. It has no sense of self or motivations or consciousness. It is awash with untapped potential. Add a few more neurons to the mix, and suddenly that potential starts clicking and firing. Add 100 billion more, and you have a busy brain—consciousness emerges, emotions are felt, and the self is defined.
“THE INTERNET SHIFTED PEOPLE'S PERSPECTIVES FROM 'ME' TO 'US'.”
—BRIAN UZZI
Since the advent of the Internet, humans have connected with increasing ease and, like a network of neurons, demonstrated different and exciting behaviors in groups. People rarely make decisions without first asking for advice from social media “friends.” They crowdsource ideas on Twitter, feedback on Facebook, and funds on Kickstarter. As seen in the image on these two pages, users—via the Internet—exchange files, movies, and music with friends and strangers all around the globe. (Read more about Luis Amaral’s BitTorrent research on page 17.) Together, they weave the fabric of the future, embellishing it with shared information and pictures along the way. “We used to think about the world from an almost entirely individual perspective,” says Brian Uzzi, co-director of the Northwestern Institute on Complex Systems (NICO). “The Internet shifted people’s perspectives from ‘me’ to ‘us.’”
FOCUSING ON COMPLEXITY
Be it neural networks or human crowds, a system’s parts do not necessarily provide insight into how the system will behave as a whole. The discipline of complexity science attempts to understand and predict the behaviors of complete systems. McCormick scientists and engineers have applied complexity theories widely from systems as small as the inner workings of a single cell to those as expansive as climate and social behaviors.
Through the lens of complexity, they can study how businesses make up an economy, how housing initiatives create segregated neighborhoods, how electrical connections contribute to the power grid, and how animals make up a food web. They seek to understand in detail how parts of these systems connect and work together and what happens when one piece fails.
At Northwestern, the complexity enterprise began with a few lunches among four professors: Julio M. Ottino, Daniel Diermeier, Uri Wilensky, and Luis Amaral. Starting in 2001, they met regularly to discuss their respective complexity research and discover ways their work intersected. Gradually, more and more faculty members joined these lunchtime discussions until there was enough inter-est to form an institute. The group established the Northwestern Institute on Complex Systems (NICO) in 2004 as a hub and facili-tator for path-breaking and relevant complexity science research that transcends the boundaries of established disciplines.
“When we formed NICO, the landscape was simmering with activity,” says Ottino, dean of McCormick. “We knew it was time to set up a formal center for complexity research. We wanted to be in the center of this new field and contribute to the development of new theory and tools.” Joining faculty from far-ranging disciplines, including foreign languages, social policy, industrial engineering, finance, and cell biology, Northwestern was the first university to establish such an institute to embrace the complex. “Some great universities have complexity meetings and seminars, but no other institution brings everyone together like NICO does,” Wilensky says. “That’s one of the reasons Northwestern stands as one of the best places in the world to study complex systems.”
Indeed, the high quality of research at NICO solidifies it as a premier complex systems institute. Adding to Northwestern’s eminence, Wilensky is the founder of NetLogo, the most-widely used agent-based modeling platform for the study of complex systems. With a need to better understand the increasing complexity and interdependence of the world, scientists use NetLogo to simulate actions and behavior in ways that previously seemed impossible. “Complexity is about knowing which kinds of predictions you can make and what types of approaches are useful to handle those predictions,” says Luis Amaral, co-director of NICO. “The basic idea is that the system is more than a sum of its parts.”
LEVERAGING NETWORKS FOR CHANGE
LUIS AMARAL
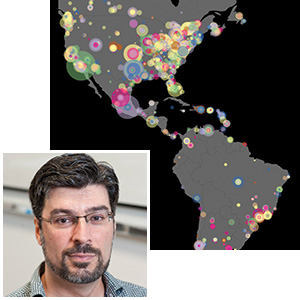
Armed with unprecedented amounts of data, Luis Amaral has applied a complexity approach to discover insights in a number of diverse areas, including Internet usage and physicians’ decisions when treating patients.
Last fall, Amaral, professor of chemical and biological engineering at McCormick and of medicine at the Feinberg School of Medicine, teamed up with Fabian E. Bustamante, professor of electrical engineering and computer science, to analyze traffic in BitTorrent, a popular file sharing system. Peer-to-peer file sharing of movies, television shows, music, and books over the Internet has surged worldwide over the past several years. Amaral and Bustamante’s study of BitTorrent usage revealed the emergence of online communities organized around common interests.
Using connection patterns gathered from 10,000 BitTorrent users during a one-month period, Amaral and Bustamante discovered two surprising behavior patterns. First, most BitTorrent users are content specialists—sharing only music or movies or books but not all three. And second, users in countries with similar levels of economic development tend to download similar types of content. For example, those living in poorer countries primarily download movies.
“Looking into this world of Internet traffic, we see a close interaction between computing systems and our everyday lives,” Amaral says. “People in a given country display preferences for certain content—content that might not be readily available because of an authoritarian government or inferior communi- cation infrastructure. This study provides a great deal of insight into how things are working in a country.”
In another study, Amaral analyzed physician social networks, examining their professional connections and methods of sharing information. He sought to understand why one physician might recommend newly developed therapies while another might choose a more traditional route. He found that doctors are more likely to recommend a new therapy to patients when persuaded to do so by an influential colleague.
A commonly accepted belief is that physicians “catch” a new therapy in what is known as a contagion model. One doctor sees another prescribing a drug or ordering a test, becomes “infected” by the new approach, and begins to implement it. Amaral’s study, however, found the art of persuasion was more effective at boosting adoption of a new approach. The trick for physicians in influencing their colleagues was finding the sweet spot in frequency and tone of persuasive messages so they’re effective and not off-putting. Along with Feinberg’s Curtis Weiss, assistant professor of pulmonary medicine, Amaral found that this sweet spot took the form of a reminder message delivered every five to seven days as a strong suggestion but not an order.
“We learned how to propagate ideas among physicians without external pressure,” Amaral says. “Instead of demanding people do something, you tell them it’s important. Then you have those people convince other peers, and it leads to a change in culture.”
MAKING COMPLEXITY VISIBLE
URI WILENSKY
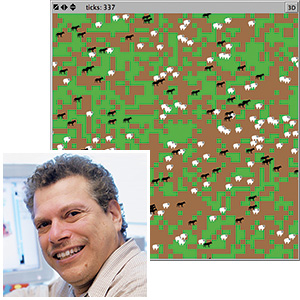
Complexity researchers everywhere are intimately familiar with Uri Wilensky’s work. He is the creator of NetLogo, the most used agent-based programming language and modeling environment in the world. Wilensky began its development in 1988 as a student at MIT, where he studied how people think and learn with tech- nology. This work lead to the creation of NetLogo, which enables users to explore and analyze the phenomena that emerge from the interactions of individuals. As NetLogo grew and evolved, Wilensky redesigned it with two seemingly conflicting goals.
“I wanted it to be easy enough for kids to use, so we could use it for education,” says Wilensky, professor of computer science and learning sciences. “And I wanted it to be sophisticated enough so that researchers would use it for their high-level investigations. A lot of people didn’t think we could achieve both goals, but I’m proud to say we did so in spades.”
As evidence, he notes that research using NetLogo has resulted in several thousand scientific articles published in top journals. At the same time, tens of thousands of students use NetLogo in school to learn about chemistry, anthropology, economics, and more. Freely available online, NetLogo includes an extensive library of models for public use. Wilensky’s new book, An Introduction to Agent-Based Modeling: Modeling Natural, Social, and Engineered Complex Systems with NetLogo, was published by MIT Press in April.
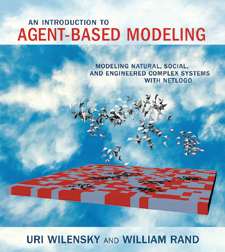
Agent-based modeling is a type of computer simulation used to examine how the behavior of systems with many interacting individuals unfolds over time. NetLogo allows users to divide phenomena into different agents and give the agents properties and rules that control their behaviors and interactions. Within the simulation, agents move and follow those rules to demonstrate how the system grows and changes over time. Agents can be anything—atoms in a material, animals in an ecosystem, or people in a community.
“You start with a seed and grow whatever you want,” Wilensky says. “All you need is something composed of parts that interact. That’s essentially what a complex system is, which makes agent-based modeling a suitable methodology for studying complexity. The trick is to figure out how to break a system down into interesting parts.”
PREDICTING CLIMATE TIPPING POINTS
MARY SILBER
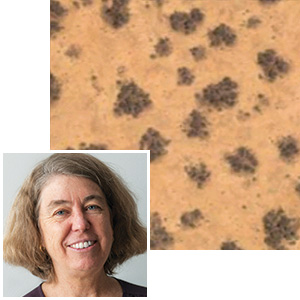
When Mary Silber looks at the desert, she sees leopards and tigers. A professor of engineering sciences and applied mathematics, Silber examines satellite images of vegetation patterns that merge in semi-arid ecosystems, including regions in Niger and Sudan. The vegetation shifts as water diminishes, forming spots and stripes that, from afar, resemble the patterns in animal fur.
Analyzing vegetation patterns is just one way Silber uses mathematics to determine whether or not it’s possible to anticipate when Earth’s climate may be on the brink of an abrupt transition.
“The thing about tipping points is that they sneak up on you,” she says. “And they can have very dramatic effects.”
As a complex system, climate has numerous interacting components, which makes modeling difficult and tipping points potentially impossible to predict—a challenge Silber has enthusiastically taken up. She and her group are developing mathematics that will identify robust and universal qualitative phenomena associated with complex systems, including climate.
Before she could work on the problem, she needed to settle on tipping point characteristics that suggest a mathematical framing: a threshold that is irreversibly crossed, creating a significant qualitative change. This fits with her extensive knowledge of bifurcation theory, a well-established mathematical framework that explains and models how a small, smooth change in a system can cause a sudden, dramatic change overall.
The semi-arid ecosystems that Silber studies appear to be nearing a dangerous threshold. Climate change may make them increasingly arid, pushing them into deserts unable to sustain life. Viewing these ecosystems through the lens of complexity, Silber examines how climate-tipping events correspond to bifurcations. She thinks pattern formation in vegetation might tell us more about the timing of sudden transitions associated with an ecosystem’s collapse under climate change. These patterns might serve as indicators that could help the world prepare for— or even prevent—going beyond the point of no return.
EXPLORING COMPONENTS OF CREATIVITY
BRIAN UZZI
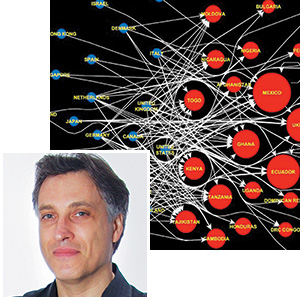
Does creativity result from a passing epiphany or a cleverly organized network?
In trying to understand what makes a piece of work creative, Brian Uzzi has taken subjects as diverse as scientific papers, Broadway musicals, and patented innovations and broken them down into their bare components. Uzzi, the Richard L. Thomas Professor of Leadership and Organizational Change in the Kellogg School of Management and, by courtesy, professor of industrial engineering and management sciences in McCormick, set out to find what mix of certain factors made a work special and new.
“Instead of spontaneously emerging from one great mind, creativity is more like an import/export business,” Uzzi says. “Creative people take things that are conventions in one field and bring them into a new field where they suddenly appear as innovative. A lot of creative work emerges that way.”
In one study, Uzzi examined scientific papers published since the 1950s and coded them for their component parts. He then tallied the components that appeared most frequently and studied the other components with which they most typically appeared. Uzzi found that the most celebrated papers contained a certain mix of new and old knowledge.
“Creative genius and potluck dinners have an essential commonality,” Uzzi says. “The biggest hits are essentially 90 percent conventional knowledge with just 10 percent of something new and different.”
ORDERING THE OPPOSITE OF CHAOS
NOSHIR CONTRACTOR
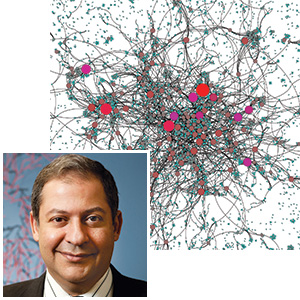
Contrary to the butterfly effect, self-organizing systems move from chaos to order. Contractor, the Jane and William White Professor of Behavioral Sciences, is interested in applying this theory to the complexity of how social structures self-order. Contractor says self-organizing systems are not stable as a result of being static; instead they are stable because they continually self-organize themselves to remain constant despite underlying chaos. He likens it to the first few weeks as a freshman at a new school.
“Initially, you randomly meet people,” Contractor says. “But in a few short weeks, your social network begins to look fairly stable. You have friends you see on a regular basis, but you have to continually do things to renew the friendship.”
Contractor builds rigorous computational models of self-organizing systems to examine how these networks evolve and stabilize. Based on simple social rules derived from well-established social science theories, these models are validating the use of empirical data. For example, Contractor has used such models to better understand how leaders emerge in teams, how online communities organize, and how new scientific disciplines arise. Most recently, NASA funded Contractor and his collaborator Leslie DeChurch from Georgia Tech to model team and task dynamics among individuals that the US government aeronautics agency plans to send on a mission to Mars.
“People who work in offices go home at the end of the day and don’t have to see their team again until the next day,” Contractor says. “Now, imagine you’re stuck in a space capsule going to Mars, living a year on Mars, and traveling a year to get back to earth. There’s no leaving the team. The dynamics are likely to be very different.”
COAXING NATURE TO WORK HARDER
MILAN MRKSICH & MICHAEL JEWETT
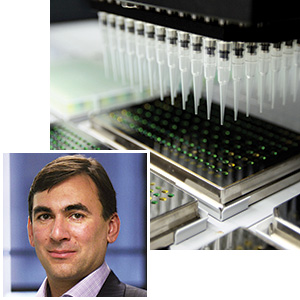
From using yeast to brew beer to producing ethanol from corn, society has long employed nature to serve its needs. Synthetic biology has taken this concept a step further by turning biological systems into small, efficient factories that manufacture chemicals too expensive and tedious to produce in the chemistry lab.
This advance, however, comes with a major challenge. Popularly used biological systems, such as cells and bacteria, have their own objectives. They want to survive, eat, and proliferate and will exhibit strong resistance when forced to perform other functions.
“When we try to engineer a cell with other enzymes, that can often lead to unwanted effects on the enzymes already there,” says Milan Mrksich, the Henry Wade Rogers Professor of Biomedical Engineering, Chemistry, and Cell and Molecular Biology. “It’s incredibly complex to make an addition to a cell while allowing all of the existing machinery to keep operating.”
Funded by Leslie and John A. “Mac” McQuown (ME ’57), Mrksich and synthetic biologist Michael Jewett are working together to better understand the properties of protein networks in a cell. By developing the first large-scale assessment of such networks, they aim to coax the cells to bypass their own needs and produce molecules of interest.
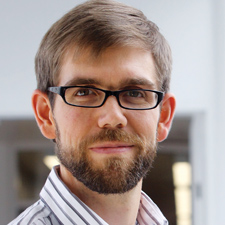
“These organisms are very complex. We want to develop new tools that allow us to design within this complexity to make many different products,” says Jewett, assistant professor of chemical and biological engineering.
The team’s research is assisted by a technology—self-assembled monolayers desorption ionization (SAMDI)—developed in Mrksich’s lab. The technology measures biochemical reactions in an extremely fast, low-cost way. SAMDI enables them to analyze thousands of cellular pathways at once while observing unintended consequences on other cellular functions.
“At the end of this, we’re going to better understand how and why nature’s designs work as they do,” Jewett says. “We’ll be able to create new design rules for constructive biology that move beyond what does exist to what can exist.”