Flexible Thinking, Expansive Ideas
John Rogers bends historically rigid ideas about electronics to create new medical monitoring and treatment devices that flex and stretch with the human body.
You see them nearly everywhere—clinging to wrists, clipped to pockets, and even embedded in most smartphones. As of 2014, one in five Americans reported owning a wearable device to monitor fitness, manage sleep, or track their heart rates. The number of users grow daily.
Now imagine what would happen if these devices went beyond just being wearable in the conventional sense—adhering directly to our skin, for example, or becoming seamlessly integrated into our bodies—with functionality that extended beyond simply monitoring fitness to providing clinical-grade health tracking and actually treating illnesses.
That is exactly what John Rogers imagines and what he’s actually bringing to life. A renowned bioelectronics pioneer, Rogers develops novel bio-integrated electronic devices that have the potential to revolutionize materials science, engineering, and medicine.
“All wearable devices that exist today use the same engineering principles,” Rogers says. “They all require a hard piece of electronics and a battery, fixed in a very clumsy way to the body with a strap or wristband, with the goal of tracking health and wellness. The measurement capabilities and the fidelity of those measurements are highly constrained, however, by the lack of stable, intimate interface to the body. As a result, these devices can provide information on ‘steps’ or ‘activity level,’ but they cannot yield clinically meaningful data.”
Bringing Bioelectronics Leadership To Northwestern
Rogers, who joined Northwestern this fall as the Louis Simpson and Kimberly Querrey Professor of Materials Science and Engineering, Biomedical Engineering and Neurological Surgery, has gained international recognition for developing bioelectronics—classes of soft, biocompatible electronic devices that can integrate seamlessly with the human body to provide a range of functions that can address challenges in clinical medicine and health.
For example, he has developed a suite of health monitoring devices that look and feel like temporary tattoos, which are radically different from conventional wearables that exploit the typical watch or wristband format. Such devices uniformly, softly adhere to the surface of the skin, collecting the person’s vital signs and then wirelessly transmitting that data to a computer or smartphone equipped with the kind of technology used in mobile financial transactions.
“Our devices can make measurements that quantitatively match those of gold-standard hospital instruments,” says Rogers, whose bendable, stretchable electronic devices also include implantable medical devices that can harvest energy from organs, wirelessly monitor biological processes, and automatically treat medical conditions, such as abnormal heart rhythms. “This is a key qualitative difference between what we’re able to do by leveraging new ideas in materials science, mechanics, and manufacturing and what is possible with wearables as they exist today.”
Rogers leads the new Center for Bio-Integrated Electronics, part of the Simpson Querrey Institute for BioNanotechnology housed in the new Louis A. Simpson and Kimberly K. Querrey Biomedical Research Center on Northwestern’s Chicago campus. Although his primary appointment is in the McCormick School of Engineering, Rogers has an additional appointment in Northwestern’s Feinberg School of Medicine.
“Invention requires expertise and creative thinking in many areas,” says Rogers, who comes to Northwestern from the University of Illinois at Urbana–Champaign. “Joining a university that really fosters interdisciplinary work, and where there are close connections between the schools of engineering and medicine in support of an active hospital and rehabilitation enterprise, was very important for us at this stage of our work.”
An Interdisciplinary, "Bell-Labsian" View
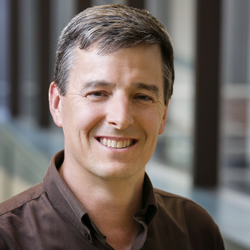
After earning a PhD at the Massachusetts Institute of Technology and serving as a postdoctoral fellow at Harvard University, Rogers joined Bell Laboratories as a technical staff member in its Condensed Matter Physics Research Department. He credits his seven years there for shaping his approach to research, which he describes as interdisciplinary, fundamental science with potential for broader consequences in engineering and technology beyond the lab.
“The Bell Labs style didn’t involve an application-constrained approach to research, but rather one of research with an eye toward potential applications,” Rogers says. “I have tried to adopt and morph that spirit for my lab. It’s a ‘Bell-Labsian’ worldview, I guess.”
MOVING BEYOND RIGID, POINT-CONTACT ELECTRODES AS INTERFACES TO THE BODY, TO FULLY INTEGRATED, SOFT FORMS OF ELECTROICS FOR DRASTICALLY INCREASED LEVELS OF FUNCTIONALITY—BLURRING THE BOUNDARIES BETWEEN BIOLOGY AND ELECTRONICS"
That mindset led Rogers to his current work in health monitoring devices. Initially interested in novel electronic materials, Rogers hoped to make integrated circuits, traditionally made of rigid silicon, more flexible—for use perhaps in roll-up displays. It wasn’t until a team of neurologists at the University of Pennsylvania suggested that these flexible electronic systems could be placed directly onto the brain and used for monitoring that he realized a compelling and much broader relevance for his work in human health.
“We had never thought about this,” Rogers says. “It placed different requirements on the materials and mechanics of our devices, but we started to think about how to build soft electronics systems and about how they might offer new ways to interface technology with the human body.”
Electronics, Not Electrodes
Researchers and physicians have used electrodes for medical purposes for hundreds of years. An electrocardiogram, for example, places electrodes on the skin to record the heart’s electrical activity and determine abnormalities. Rogers aimed to take this basic idea dramatically forward to a new frontier.
“The idea of taking rigid, integrated circuit technology and reformulating it—from the bottom up—to allow it to softly wrap around the surface of the brain, meld onto the skin, or form around a beating heart—nobody was doing that,” Rogers says. “That’s where we saw an opportunity. Moving beyond rigid, point-contact electrodes as interfaces to the body, to fully integrated, soft forms of electronics for drastically increased levels of functionality—blurring the boundaries between biology and electronics.”
Rogers and his team discovered that silicon becomes naturally flexible when it’s extremely thin. They bonded thin silicon in a wavy geometry to a soft, wavy rubber substrate, which enabled the silicon to twist, bend, and stretch like a rubber band. This set of capabilities opened the door for advanced devices to monitor—and even treat—the heart and brain.
For example, Rogers and longtime collaborator Yonggang Huang, Walter P. Murphy Professor of Mechanical Engineering and Civil and Environmental Engineering at Northwestern, developed an elastic silicon membrane capable of steadying an irregular heartbeat. Such mechanical, electrical, and biomedical feats have earned Rogers a series of prestigious accolades, including a MacArthur Fellowship, the Lemelson-MIT Prize, and election into the National Academy of Engineering and the National Academy of Sciences.
From The Cradle
Rogers’s skin-mounted health monitoring device is now being tested in the neonatal intensive care unit (NICU) at Lurie Children’s Hospital of Chicago. His wireless, battery-free, temporary “tattoo” can monitor all vital signs of the most fragile humans in a much gentler way than traditional monitors. Just this summer, Rogers’s team finished two rounds of successful testing with human babies in collaboration with Amy Paller, professor of dermatology in the Feinberg School of Medicine.
“If you go into a NICU today, you will see very tiny babies all hooked up with tape and hard-wire connections to external monitoring electronics,” Rogers says. “Even the least-adhesive tapes can cause permanent scarring because a baby’s skin can be extremely fragile. The wires represent significant mechanical constraints on natural motions. We can get rid of all this hardware and achieve the same kind of measurement functionality with thin, skin-like, wireless, battery-free systems, which also enable the kind of mother-baby interactions that are critically important to healthy development.”