News
Designing Way to Potentially Make Oxygen Injectable
September 2nd, 2022
What if emergency medical personnel could treat a desperately ill patient in need of oxygen with a simple injection instead of having to rely on mechanical ventilation or rush to get them onto a heart-lung bypass machine?
Now, a new approach to transport gases using a class of materials called porous liquids represents a big step toward artificial oxygen carriers and demonstrates the immense biomedical potential of these unusual fluids.
In a study recently published in Nature, a Harvard University-led team of scientists that also included Northwestern Engineering’s Monica Olvera de la Cruz detail a new approach to transporting gases in aqueous environments using porous liquids. The authors identified and tailored multiple porous frameworks that can store much higher concentrations of gases, including oxygen and carbon dioxide, than normal aqueous solutions. This breakthrough may hold the key to creating injectable sources of oxygen as a bridge therapy for cardiac arrest, creating artificial blood substitutes, and overcoming longstanding challenges in preserving organs for transplants.
“We realized that there would be a lot of benefits to using liquids with permanent microporosity to address gas transport challenges in water and other aqueous environments,” said Jarad Mason, the paper’s senior author and assistant professor of chemistry and chemical biology at Harvard. “We’ve designed fluids that can transport oxygen at densities that exceed that of blood, which opens up exciting new opportunities for transporting gases for a variety of biomedical and energy applications.”
Liquids with permanent microporosity are a new class of materials that are composed of microscopic porous particles dispersed in a liquid medium. Imagine tiny recyclable sponge-like bits capable of soaking up gases in its holes and releasing them. Until now, all porous liquids have consisted of microporous nanocrystals or organic cage molecules dispersed in organic solvents or ionic liquids that are too large to diffuse through the pore entrances. The researchers developed a new strategy to create aqueous porous liquids—termed “microporous water”—with high gas capacities based on thermodynamics.
Read the full article here
Story written by Yahya Chaudhry, and originally published in McCormick Engineering News
Controlling the Shape of Chiral Molecules
August 16th, 2022
Common in nature and found in DNA and amino acids, chiral molecules are asymmetric with a structure that’s different from its mirror image. Chiral amino acids are “left-handed” and cannot be swapped for a “right-handed” equivalent. Why these molecules are lefties is unknown. It’s also unclear how chirality at the molecular scale translates into the much larger meso-scale.
Looking at the chirality of molecules attracted to both water and fats, otherwise known as amphiphiles, a team of Northwestern Engineering researchers led by Michael Bedzyk and Monica Olvera de la Cruz analyzed how to organize the molecules into bigger units. The study showed how different shapes of chiral molecules can be created, which is key information because the utility of chiral molecular assemblies in drug delivery and nanoelectronics is coupled to their overall shape and internal architecture.
“The utility of molecular assemblies for biosensing, drug delivery, and nanoelectronics is sensitively connected to the assemblies’ overall shape and internal molecular packing or structure,” Bedzyk said. “This study shows how to attain and control chiral membrane shapes by tuning the solution environment, which should be useful for varied applications based on chiral building blocks.”
Bedzyk, Olvera de la Cruz, and their collaborators presented their work in the paper “Electrostatic Control of Shape Selection and Nanoscale Structure in Chiral Molecular Assemblies,” published August 2 in the journal ACS Central Science. Bedzyk is a professor of Materials Science and Engineering and Physics and Astronomy, and Olvera de la Cruz is the Lawyer Taylor Professor of Materials Science and Engineering, Chemistry, and Chemical and Biological Engineering at Northwestern Engineering.
Read the full article here.
Story written by Brian Sandalow, and originally published in McCormick Engineering News
Upcycling Polyester Could Reduce Plastic Waste
March 21st, 2022
Less than 10 percent of the 380 million metric tons of annually produced plastics are currently recycled in the US, creating a global environmental threat caused by plastic production and the absorption of millions of tons of waste into bodies of water each year. When you look at plastics like polyester, that percentage goes down.
Instead of renewing a commitment to recycling techniques that can only be used once on certain plastics, researchers have looked to other solutions to reduce plastic waste. Plastic upcycling, the process of efficiently deconstructing and rebuilding polymers, which are the essential building blocks of plastic, has become a major area of focus.
Now, a Northwestern Engineering-led team has provided the basis for a technique to enhance the effects of an enzyme that breaks down polyester — the plastic used to make soda bottles and inexpensive clothing (and commercially termed PET) — into its fundamental parts, a development that could help engineers develop solutions for removing microplastics from rivers and oceans.
The McCormick School of Engineering’s Monica Olvera de la Cruz, the senior author of the paper, explained that in recycling, plastic is heated up, broken down and then rebuilt into weaker, poorer quality plastics. But in upcycling, breaking down polymers into their fundamental components sometimes allows them to become even sturdier plastics than they were before.
Olvera de la Cruz’s team hoped to create as green an upcycling process as possible; one that didn’t create pollutants but instead removed them. Using an enzyme that can be synthesized in a lab, the researchers developed a process without using other solvents that can be used repeatedly.
Read the full article here.
Story written by Win Reynolds, and originally published in McCormick Engineering News
How Understanding Metallization of Materials Could Help the Development of Superionic Materials for Safe, Efficient Batteries
November 19th, 2021
Suitable solid-state ionic conductors are a key to producing new safe and efficient batteries. Candidate materials for solid state batteries are superionics, which show a transition from an insulating state to a conducting state in which one ionic species is mobile.
Recent work from Northwestern Engineering could make it easier to study properties of these superionic materials.
Led by Monica Olvera de la Cruz, Lawyer Taylor Professor of Materials Science and Engineering, a team of McCormick School of Engineering researchers observed a classical system that exhibits a transition between insulating and conducting states. The group hopes this will accelerate the development of safe solid-state batteries, with the usage of metallizable colloidal crystals for future prototypes.
The paper “Metallization of Colloidal Crystals” was published November 11 in the academic journal Physical Review Materials.
The transition between insulating and conducting states is called “metallization” because the mobile ions in the compound behave like electrons in metals. Using computational and numerical analysis, Olvera de la Cruz and her colleagues found related insulator-like to metal-like transitions in size-asymmetric binary colloidal mixtures. The components of the binary colloidal crystals they studied are much larger than atoms and electrons, and their arrangements can be observed in optical microscopes. Therefore, the researchers avoided using the mathematical framework that describes atoms and electrons – quantum mechanics – and instead employed classical mechanics to provide a more general explanation of the transition.
The investigators found that vibrations of the larger and mostly static species enable the mobility of the smaller colloidal particles. This process has a subatomic analog in which coordinated motion of vibrating protons gives way to conducting electrons, which is seen in some atomic materials exhibiting an insulator-to-metal transition. The research team’s observation of a metallization transition in a system with no charge is important given that these systems are not limited by charge conservation laws and therefore are highly tunable.
Read the full article here.
Story written by Brian Sandalow, and originally published in the McCormick Engineering News
Kalow and Olvera de la Cruz Receive Five-Year NSF Award Grant
September 13, 2021
National Science Foundation has awarded a five-year, $20 million grant to researchers at Northwestern and eight other institutions to explore and optimize the chemical structure and physical properties of individual molecules in a polymer network.
“The long-term potential of this research includes cost- and time-efficient optimization of the polymers used in products like biomedical implants, building materials and even automobile tires,” said principal investigator Stephen Craig, the William T. Miller distinguished professor of chemistry at Duke University. “We hope to discover new ways to make tougher, longer-lived materials with improved end-of-life properties that reduce waste while being perfectly tailored to their intended uses.”
The NSF Center for the Chemistry of Molecularly Optimized Networks (MONET) will bring together experts in polymer chemistry, synthetic methods, photochemistry, multi-scale modeling and bioconjugation. While led by Duke, the center will include researchers from Columbia University, Johns Hopkins, MIT, Northwestern, University of California - San Diego, University of Illinois Urbana-Champaign, University of Michigan, and University of Washington.
The senior investigator team includes Northwestern’s Julia Kalow, Assistant Professor of Chemistry, who develops dynamic polymer networks, and Monica Olvera de la Cruz, the Lawyer Taylor Professor of Materials Science and Engineering and Chemistry, a soft-matter theorist.
“The scientific path being charted by the MONET team will further national priorities in Advanced Manufacturing, Sustainability and Artificial Intelligence,” said NSF Division Director David Berkowitz.
In addition to its scientific activities, MONET will work to broaden participation and improve student training in the sciences and advance the public appreciation and commercial translation of its findings.
Congratulations Professor Kalow and Professor Olvera de la Cruz!
originally published September 8, 2021 in Department of Chemistry News
Microspheres Quiver When Shocked
Columbia and Northwestern engineers use electric fields to induce oscillations in tiny particles; this motion could be used by researchers to develop microbots
June 23, 2021 | By Columbia University School of Engineering and Applied Science
Newswise — New York, NY—June 23, 2021—A challenging frontier in science and engineering is controlling matter outside of thermodynamic equilibrium to build material systems with capabilities that rival those of living organisms. Research on active colloids aims to create micro- and nanoscale “particles” that swim through viscous fluids like primitive microorganisms. When these self-propelled particles come together, they can organize and move like schools of fish to perform robotic functions, such as navigating complex environments and delivering “cargo” to targeted locations.
A Columbia Engineering team led by Kyle Bishop, professor of chemical engineering, is at the forefront of studying and designing the dynamics of active colloids powered by chemical reactions or by external magnetic, electric, or acoustic fields. The group is developing colloidal robots, in which active components interact and assemble to perform dynamic functions inspired by living cells.
In a new study published today by Physical Review Letters, Bishop’s group, working with collaborators at Northwestern University’s Center for Bio-Inspired Energy Science (CBES), report that they have demonstrated the use of DC electric fields to drive back-and-forth rotation of micro-particles in electric boundary layers. These particle oscillators could be useful as clocks that coordinate the organization of active matter and even, perhaps, orchestrate the functions of micron-scale robots.
“Tiny particle oscillators could enable new types of active matter that combine the swarming behaviors of self-propelled colloids and the synchronizing behaviors of coupled oscillators,” says Bishop. “We expect interactions among the particles to depend on their respective positions and phases, thus enabling richer collective behaviors—behaviors that can be designed and exploited for applications in swarm robotics.”
Read the full article here
Mimicking shapes found in bacteria
For first time, researchers control design of bacterial microcompartments
April 28, 2021 | By Amanda Morris
Bad bacteria can survive in extremely hostile environments — including inside the highly acidic human stomach — thanks to their ability to sequester toxins into tiny compartments.
In a new study, Northwestern University researchers have, for the first time, theoretically and experimentally controlled protein assembly to build these microcompartments into different shapes and sizes, including long tubes and polyhedra. Not only does this work illuminate how biological units, such as viruses and organelles, develop, it also could inform new ways to design medicine, synthetic cells and nanoreactors.
Further down the road, these insights potentially could lead to new antibiotics that target microcompartments of pathogens while sparing good bacteria.
“By carefully designing proteins to have specific mutations, we were able to control assembly of the proteins that form bacterial microcompartments,” said Northwestern’s Monica Olvera de la Cruz, who led the theoretical computation. “We used this also to predict other possible formations that have not yet been observed in nature.”
“Based on previous observations, we have known that the geometry of microcompartments can be altered,” added Danielle Tullman-Ercek, who led the experimental work. “But our work provides the first clues into how to alter them to achieve specific shapes and sizes.”
Olvera de la Cruz is the Lawyer Taylor Professor of Materials Science and Engineering at Northwestern’s McCormick School of Engineering. Tullman-Ercek is an associate professor of chemical and biological engineering at McCormick.
The research is featured in the April issue of ACS Central Science, which published today (April 28).
Read the full article on Northwestern Now.
Surprise in Nanoparticle Aggregation Improves Rational Design of Macromolecular Structures
Predicting how particles experience changes in charge when they form clusters could markedly improve the accuracy of computational techniques used in drug discovery and in the design of nanomaterials
March 31, 2021 | By Brian Sandalow
Accurate prediction of macromolecular structure and function is a key challenge in many fields, from designing new nanomaterials to drug discovery. New counterintuitive findings from Northwestern Engineering could significantly bolster the accuracy of computational structure prediction methods, helping the development of new drugs and nanomaterials.
Erik Luijten and Tine Curk, a postdoctoral researcher in Luijten’s team, found that when two or more identical nanoparticles, proteins, or other biomolecules come together, there’s a spontaneous and global redistribution of charge that can cause initially matching particles to take on different charges in a cluster. The collective effect can qualitatively change the structure of nanoscale systems.
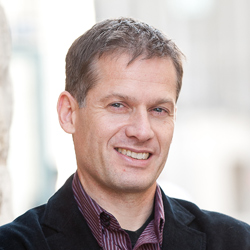
Erik Luijten
Importantly, this finding shows that charge regulation – the mechanism responsible for the change in particle charge – cannot be ignored when making predictions about how the structure will react.
“This teaches us that global-charge redistribution must be accounted for to accurately predict structure formation of molecular and nanoscale systems,” said Luijten, professor and chair of the materials science and engineering department at the McCormick School of Engineering.
Read the full article here
Designing Soft Materials that Mimic Biological Functions
Soft material demonstrates autonomous, heartbeat-like oscillating properties
Mar 1, 2021 |
Aquatic robot inspired by sea creatures walks, rolls, transports cargo
Soft material is powered by light and rotating magnetic fields
December 09, 2020 | By Amanda MorrisNorthwestern University researchers have developed a first-of-its-kind life-like material that acts as a soft robot. It can walk at human speed, pick up and transport cargo to a new location, climb up hills and even break-dance to release a particle.
Nearly 90% water by weight, the centimeter-sized robot moves without complex hardware, hydraulics or electricity. Instead, it is activated by light and walks in the direction of an external rotating magnetic field.
Resembling a four-legged octopus, the robot functions inside a water-filled tank, making it ideal for use in aquatic environments. The researchers imagine customizing the movements of miniature robots to help catalyze different chemical reactions and then pump out the valuable products. The robots also could be molecularly designed to recognize and actively remove unwanted particles in specific environments, or to use their mechanical movements and locomotion to precisely deliver bio-therapeutics or cells to specific tissues.
“Conventional robots are typically heavy machines with lots of hardware and electronics that are unable to interact safely with soft structures, including humans,” said Samuel I. Stupp, who led the experimental research. “We have designed soft materials with molecular intelligence to enable them to behave like robots of any size and perform useful functions in tiny spaces, underwater or underground.”
“By combining walking and steering motions together, we can program specific sequences of magnetic fields, which remotely operate the robot and direct it to follow paths on flat or inclined surfaces,” added Monica Olvera de la Cruz, who led the theoretical work. “This programmable feature allows us to direct the robot through narrow passages with complex routes.”
The research was published today (Dec. 9) in the journal Science Robotics.
Stupp is Board of Trustees Professor of Materials Science and Engineering, Chemistry, Medicine and Biomedical Engineering at Northwestern. He has appointments in the McCormick School of Engineering, Weinberg College of Arts and Sciences and Feinberg School of Medicine. Olvera de la Cruz is the Lawyer Taylor Professor of Materials Science and Engineering, Chemistry and Chemical and Biological Engineering in McCormick.
Stupp and Olvera de la Cruz also are the director and deputy director, respectively, of the Center for Bio-Inspired Energy Science, a Northwestern-based Energy Frontier Research Center funded by the U.S. Department of Energy.
New advances
The study builds on Stupp’s previous work to design “robotic soft matter” that imitates living sea creatures. In the previous study, published earlier this year, the robotic material could bend over time scales of minutes and crawl on a surface by taking one step every 12 hours. Now, the current breakthrough allows the robot to walk with human speed — about one step per second — and respond to magnetic fields that steer these materials to follow specific trajectories.
By coupling responses to light and magnetic fields, the researchers designed a robot that also can pick up cargo and deliver it to a destination by walking or rolling. Then it drops the cargo at the new location by either inverting its shape — allowing smooth payloads to gently slide off the robot — or performing a spinning “break dance” to dislodge and release stickier objects.
“The design of the new materials that imitate living creatures allows not only a faster response but also the performance of more sophisticated functions,” Stupp said. “We can change the shape and add legs to the synthetic creatures, and give these life-like materials new walking gaits and smarter behaviors. This makes them highly versatile and amenable to different tasks.”
How it works
The secret to the robot’s precise movement and agility lies within its water-filled structure and the embedded skeleton of aligned nickel filaments that are ferromagnetic. The soft component is a molecularly designed network with parts that allow it to respond to light, hold or expel water in its interior, and have just the right stiffness to respond rapidly to magnetic fields.
The Northwestern team used chemical synthesis to program the molecules within the hydrogel to respond to light. When exposed to light, the robot’s molecules become hydrophobic (repelling water), causing the water molecules to escape. This conversion causes the robot to “come alive” by bending from a flat position to “standing.” The researchers discovered that this bending enables the material to respond rapidly to rotating magnetic fields, activating its ability to walk fast. When the light turns off, the molecules revert back to their original state and the robot goes flat, but it is ready anytime for a new cycle of activity under a magnetic field when prompted by an LED.
When exposed to rotating magnetic fields, the embedded skeleton in the bent robot exerts cyclic forces on the soft molecular network and activates the legs. The rotating field can be programmed to navigate the robot along a pre-determined path.
“Using theory and computation, we can calculate the response to light and magnetic fields,” Olvera de la Cruz said. “This allows us to predict and program walking trajectories with great accuracy.”
Future applications
Stupp and Olvera de la Cruz imagine these soft robotic materials potentially could be used to create objects for many applications, including chemical production, new tools for environmentally important technologies or as smart biomaterials for highly advanced medicine.
“Eventually, we’d like to make armies of microrobots that could perform a complicated task in a coordinated way,” Stupp said. “We can tweak them molecularly to interact with one another to imitate swarming of birds and bacteria in nature or schools of fish in the ocean. The molecular versatility of the platform could lead to applications that have not been conceived at this point.”
The study, “Fast and programmable locomotion of hydrogel-metal hybrids under light and magnetic fields,” was supported by the Center for Bio-Inspired Energy Science, an Energy Frontier Research Center funded by the U.S. Department of Energy Office of Basic Energy Sciences (award number DE-SC0000989).
Postdoctoral fellow Chuang Li and graduate student Garrett Lau of the Stupp lab and Hang Yuan, a graduate student in the Olvera de la Cruz lab, are the primary authors of the paper. Other coauthors include Northwestern faculty members Danna Freedman and Pedram Khalili.
A New Understanding of Ionic Interactions with Graphene and Water
Findings could inform design of environmental technologies behind water purification processes and electric energy storage
November 17, 2020 | Alex Gerage | News Link
A research team led by Northwestern engineers and Argonne National Laboratory researchers have uncovered new findings into the role of ionic interaction within graphene and water. The insights could inform the design of new energy-efficient electrodes for batteries or provide the backbone ionic materials for neuromorphic computing applications.
Known for possessing extraordinary properties, from mechanical strength to electronic conductivity to wetting transparency, graphene plays an important role in many environmental and energy applications, such as water desalination, electrochemical energy storage, and energy harvesting. Water-mediated electrostatic interactions drive the chemical processes behind these technologies, making the ability to quantify the interactions between graphene, ions, and charged molecules vitally important in order to design more efficient and effective iterations.
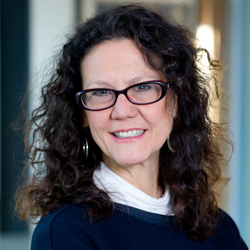
Monica Olvera de la Cruz
“Every time you have interactions with ions in matter, the medium is very important. Water plays a vital role in mediating interactions between ions, molecules, and interfaces, which lead to a variety of natural and technological processes,” said Monica Olvera de La Cruz, Lawyer Taylor Professor of Materials Science and Engineering, who led the research. “Yet, there is much we don’t understand about how water-mediated interactions are influenced by nanoconfinement at the nanoscale.”
Using computer model simulations at Northwestern Engineering and x-ray reflectivity experiments at Argonne, the research team investigated the interaction between two oppositely charged ions in different positions in water confined between two graphene surfaces. They found that the strength of the interaction was not equivalent when the ions’ positions were interchanged. This break of symmetry, which the researchers’ dubbed non-reciprocal interactions, is a phenomenon not previously predicted by electrostatic theory.
The researchers also found that the interaction between oppositely charged ions became repulsive when one ion was inserted into the graphene layers, and the other was absorbed at the interface.
“From our work, one can conclude that the water structure alone near interfaces cannot determine the effective electrostatic interactions between ions,” said Felipe Jimenez-Angeles, senior research associate in Northwestern Engineering’s Center for Computation and Theory of Soft Materials and a lead author on the study. “The non-reciprocity we observed implies that ion-ion interactions at the interface do not obey the isotropic and translational symmetries of Coulomb's law and can be present in both polarizable and non-polarizable models. This non-symmetrical water polarization affects our understanding of ion-differentiation mechanisms such as ion selectivity and ion specificity.”
“These results reveal another layer to the complexity of how ions interact with interfaces,” said Paul Fenter, a senior scientist and group leader in the Chemical Sciences and Engineering Division at Argonne, who led the study’s x-ray measurements using Argonne’s Advanced Photon Source. “Significantly, these insights derive from simulations that are validated against experimental observations for the same system.”
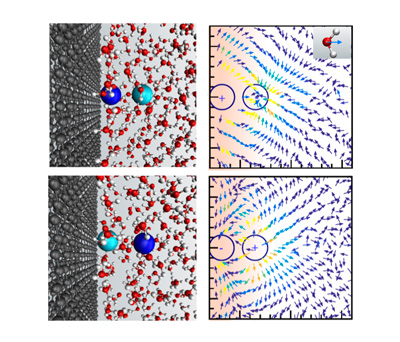
Illustration showing the interaction between ions at graphene-water interface.
These results could influence the future design of membranes for selective ion adsorption used in environmental technologies, like water purification processes, batteries and capacitors for electric energy storage, and the characterization of biomolecules, like proteins and DNA.
Understanding ion interaction could also impact advances in neuromorphic computing — where computers function like human brains to perform complex tasks much more efficiently than current computers. Lithium ion can achieve plasticity, for example, by being inserted in or removing from graphene layers in neuromorphic devices.
“Graphene is an ideal material for devices that transmit signals via ionic transport in electrolytes for neuromorphic applications,” said Olvera de la Cruz. “Our study demonstrated that the interactions between intercalated ions in the graphene and physically adsorbed ions in the electrolyte is repulsive, affecting the mechanics of such devices.”
The study provides researchers with a fundamental understanding of the electrostatic interactions in aqueous media near interfaces that go beyond water’s relationship with graphene, which is crucial for studying other processes in the physical and sciences.
“Graphene is a regular surface, but these findings can help explain electrostatic interactions in more complex molecules, like proteins,” said Jimenez-Angeles. “We know that what’s inside the protein and the electrostatic charges outside of it matters. This work gives us a new opportunity to explore and look at these important interactions.”
A paper describing the work, titled “Non-Reciprocal Interactions Induced by Water in Confinement,” was published November 17 in the journal Physical Review Research.
‘Like a fishing net,’ nanonet collapses to trap drug molecules
Novel method presents possibilities for rapidly making and testing vaccine formulations
October 05, 2020 | Amanda Morris | News Link
Northwestern University researchers are casting a net for nanoparticles.
The team has discovered a new, rapid method for fabricating nanoparticles from a simple, self-assembling polymer. The novel method presents new possibilities for diverse applications, including water purification, diagnostics and rapidly generating vaccine formulations, which typically require many different types of molecules to be either captured or delivered at the same time.
Using a polymer net that collapses into nanoscale hydrogels (or nanogels), the method efficiently captures over 95% of proteins, DNA or small molecule drugs — alone or in combinations. By comparison, loading efficiency is typically between 5% and 20% for other nanoparticle delivery systems.
“We use a polymer that forms a wide net throughout an aqueous solution,” said Northwestern’s Evan A. Scott, who led the study. “Then we induce the net to collapse. It collects anything within the solution, trapping therapeutics inside of nanogel delivery vehicles with very high efficiency.”
“It works like a fishing net, which first spreads out due to electrostatic repulsion and then shrinks upon hydration to trap ‘fish,’” added Fanfan Du, a postdoctoral fellow in Scott’s laboratory.
The paper was published last week (Sept. 29) in the journal Nature Communications.
Scott is the Kay Davis Professor of Biomedical Engineering at Northwestern’s McCormick School of Engineering. Northwestern professors Monica Olvera de la Cruz and Vinayak Dravid coauthored the paper.
Molecules found in nature, such as DNA and peptides, can rapidly self-assemble and organize into diverse structures. Mimicking this process using human-made polymer systems, however, has remained limited. Previously developed processes for self-assembling drug delivery systems are time consuming, labor intensive and difficult to scale. The processes also tend to be woefully inefficient, culminating in a small fraction of the drug actually making it inside the delivery system.
“Clinical application of self-assembled nanoparticles has been limited by difficulties with scalability and with loading large or multiple therapeutics, especially proteins,” Scott said. “We present a highly scalable mechanism that can stably load nearly any therapeutic molecule with high efficiency.”
Scott’s team found success by using a polypropylene sulfone (PPSU) homopolymer, which is highly soluble in dimethylsulfoxide (DMSO) solution, but forms electrostatic and hydrophilic aggregates in water. The aggregates are amphiphilic, which causes them to assemble into networks and eventually collapse into gels.
“Adding more water induces the network to collapse, leading to the formation of nanogels,” Du said. “The manner in which water is added affects the PPSU chain formation, which changes the nanogels’ size and structure.”
Atomistic simulations — performed by Baofu Qiao in the Olvera de la Cruz group — confirmed that the nanostructures were stabilized by weak sulfone-sulfone bonding. Using coarse-grained simulations performed by Northwestern postdoctoral fellow Trung Dac Nguyen, the researchers observed the nanonet structures. This opens a new avenue for soft materials assembly by means of sulfone-sulfone bonding.
In addition to drug delivery applications, the researchers also believe the novel method could be used for water purification. The network could collapse to collect contaminants in water, leaving pure water behind.
Scott is a member of Northwestern’s Simpson Querrey Institute, Chemistry of Life Processes Institute, Interdisciplinary Biological Sciences Program and Northwestern University Robert H. Lurie Comprehensive Cancer Center. He has a secondary appointment in the department of microbiology-immunology at the Feinberg School of Medicine.
The paper, “Homopolymer self-assembly of poly(propylene sulfone) hydrogels via dynamic noncovalent sulfone-sulfone bonding,” was supported by the Department of Energy (award number DE-FG02-08ER46539), the National Institutes of Health (grant number 1DP2HL132390-01), the National Institute of Allergy and Infectious Diseases (grant number 5R21AI137932-02), the National Science Foundation CAREER Award (grant number 1453576), the Louis A. Simpson and Kimberly K. Querrey Center for Regenerative Nanomedicine Catalyst Award and the Sherman Fairchild Foundation.
Research exposes new vulnerability in SARS-CoV-2
Electrostatic interactions enhance the spike protein’s bond to host cells
August 11, 2020 | By Amanda Morris
Northwestern University researchers have uncovered a new vulnerability in the novel coronavirus’ infamous spike protein — illuminating a relatively simple, potential treatment pathway.
The spike protein contains the virus’ binding site, which adheres to host cells and enables the virus to enter and infect the body. Using nanometer-level simulations, the researchers discovered a positively charged site (known as the polybasic cleavage site) located 10 nanometers from the actual binding site on the spike protein. The positively charged site allows strong bonding between the virus protein and the negatively charged human-cell receptors.
Leveraging this discovery, the researchers designed a negatively charged molecule to bind to the positively charged cleavage site. Blocking this site inhibits the virus from bonding to the host cell.
“Our work indicates that blocking this cleavage site may act as a viable prophylactic treatment that decreases the virus’ ability to infect humans,” said Northwestern’s Monica Olvera de la Cruz, who led the work. “Our results explain experimental studies showing that mutations of the SARS-CoV-2 spike protein affected the virus transmissibility.”
The research was published online last week in the journal ACS Nano.
Olvera de la Cruz is the Lawyer Taylor Professor of Materials Science and Engineering in Northwestern’s McCormick School of Engineering. Baofu Qiao, a research assistant professor in Olvera de la Cruz’s research group, is the paper’s first author.
Made up of amino acids, SARS-CoV-2’s polybasic cleavage sites have remained elusive since the COVID-19 outbreak began. But previous research indicates that these mysterious sites are essential for virulence and transmission. Olvera de la Cruz and Qiao discovered that polybasic cleavage site is located 10 nanometers from human cell receptors — a finding that provided unexpected insight.
“We didn’t expect to see electrostatic interactions at 10 nanometers,” Qiao said. “In physiological conditions, all electrostatic interactions no longer occur at distances longer than 1 nanometer.”
“The function of the polybasic cleavage site has remained elusive,” Olvera de la Cruz said. “However, it appears to be cleaved by an enzyme (furin) that is abundant in lungs, which suggests the cleavage site is crucial for virus entry into human cells.”
With this new information, Olvera de la Cruz and Qiao next plan to work with Northwestern chemists and pharmacologists to design a new drug that could bind to the spike protein.
Olvera de la Cruz is a member of Northwestern's Chemistry of Life Processes Institute.
The research, “Enhanced binding of SARS-CoV-2 spike protein to receptor by distal polybasic cleavage sites,” was supported by the U.S. Department of Energy (award number DE-FG02-08ER46539), the Sherman Fairchild Foundation and the Center for Computation and Theory of Soft Materials at Northwestern University.
From Ribbon to Scroll: Gaining Shape Control by Electrostatics
Controlling scroll-like cochleate structures could inform future drug-delivery strategies
Oct 14, 2019 | Alex Gerage
Northwestern Engineering materials science researchers have uncovered new insights into how electrostatic interactions can be regulated to attain and control scroll-like cochleate structures, which could inform how to capture and release macromolecules in a size-selective manner as part of future drug-delivery strategies.
Charged molecules, such as DNA and proteins, are present throughout biological systems. Membranes, a bilayer of these charged lipid molecules, are used to compartmentalize matter in a variety of structural forms, from spherical vesicles to helical nanoribbons to cochleates.
“In biology, molecules take the form of many coexisting shapes. Some are decided based on the variations placed upon them, such as concentrations of pH or salt,” said Monica Olvera de la Cruz, Lawyer Taylor Professor of Materials Science and Engineering at the McCormick School of Engineering.
“Using a simple charged biomolecule, we have shown how the interplay between electrostatic, elastic, and interfacial energies can lead to structural polymorphism, or coexistence of multiple shapes. While cochleate structures have been observed in other systems, the entire pathway for their formation had not been explained,” she added.
The team’s findings were published in a paper, titled “Electrostatic Shape Control of a Charged Molecular Membrane from Ribbon to Scroll,” on October 14 in Proceedings of the National Academy of Sciences. Olvera de la Cruz was the study’s co-corresponding author along with Michael Bedzyk, professor of materials science and engineering.
Continue reading the full article on Engineering News.
Electron-behaving nanoparticles rock current understanding of matter
Discovery will lead to new methods for materials design
June 20, 2019 | By Amanda Morris
It’s not an electron. But it sure does act like one.
Northwestern University researchers have made a strange and startling discovery that nanoparticles engineered with DNA in colloidal crystals — when extremely small — behave just like electrons. Not only has this finding upended the current, accepted notion of matter, it also opens the door for new possibilities in materials design.
“We have never seen anything like this before,” said Northwestern’s Monica Olvera de la Cruz, who made the initial observation through computational work. “In our simulations, the particles look just like orbiting electrons.”
With this discovery, the researchers introduced a new term called “metallicity,” which refers to the mobility of electrons in a metal. In colloidal crystals, tiny nanoparticles roam similarly to electrons and act as a glue that holds the material together.
“This is going to get people to think about matter in a new way,” said Northwestern’s Chad Mirkin, who led the experimental work. “It’s going to lead to all sorts of materials that have potentially spectacular properties that have never been observed before. Properties that could lead to a variety of new technologies in the fields of optics, electronics and even catalysis.”
The paper will publish Friday, June 21 in the journal Science.
Continue reading the full article on Northwestern News.
Researchers Find Enzymes Naturally Select their Own Polymer Sequences
Findings could impact fields like pharmaceutical development and industrial waste processing
Jun 11, 2018 | Danny Smith | News Link
As free-flowing enzymes travel amid a sea of polymers, a Northwestern Engineering team has found those enzymes prefer to join certain polymer sequences over others, a discovery that could lead to applications in a diverse array of fields ranging from nuclear waste processing to drug delivery.
“From all these batches of randomness, we discovered each particular enzyme selects a sequence it likes best,” said Monica Olvera de la Cruz, the Lawyer Taylor Professor of Materials Science and Engineering at Northwestern’s McCormick School of Engineering and Applied Science, who led the study. “That’s important because it sheds light on how we might design the composition of a batch of polymers, so they will disperse enzymes actively in non-biological environments.”
Authored by Olvera de la Cruz and two colleagues in McCormick’s Department of Materials Science and Engineering — senior research associate Trung Dac Nguyen and research assistant professor Baofu Qiao — the study, titled “Efficient encapsulation of proteins with random copolymers,” published today, June 11, in the Proceedings of the National Academy of Sciences.
Enzymes play a vital role in a range of chemical and biological processes by facilitating and directing biochemical reactions. Due to the limited solubility of some substrates in water, preserving and/or enhancing the catalytic capability of some enzymes in non-aqueous solutions is of increasing demand. However, most enzymes rapidly lose their chemical activity when exposed to non-biological environments, including organic solvents like toluene and tetrahydrofuran. While numerous enzyme stabilization strategies have been employed such as reverse engineering enzyme sequences, decorating enzymes with surfactants, or modifying the solvents, most of them are either limited to specific enzymes and solvents or cost ineffective.
Performing computer simulations at Quest, Northwestern’s high-powered computational facility, the researchers examined: the key factors that determined the coverage of the random copolymers with different types of enzymes in a given solvent; how the enzymes selected the random copolymers to protect themselves from unfavorable solvents; and the relationship between the enzyme surface characteristics and the polymer features.
“We found that the enzymes indeed select certain polymer sequences that best cover their surface out of the pool of the polymers,” Trung said. “The random copolymers provide the composition and sequence diversity similar to that in disordered enzymes, which explains why they can efficiently cover numerous enzymes in different size, shape, and surface patterns.”
The study, fueled by a grant from the US Department of Energy and support from the Sherman Fairchild Foundation that enabled the computational work, highlights that this special family of copolymers is an excellent candidate material for synthesizing membraneless organelles — the micron-sized liquid droplets inside cells of living organisms — as well as for stabilizing and delivering enzymes across multiple non-biological media.
“Right now, for instance, researchers in the pharmaceutical industry are trying to match sequences perfectly,” Olvera de la Cruz said. “Our discovery provides guidelines to make the dispersion of enzymes much more cost-effective and efficient.”
Olvera de la Cruz and her colleagues now plan to investigate how the membraneless organelles might spontaneously form with these copolymers-enzymes, how to control their sizes, and how the structural properties of the enzymes might be affected inside the organelles.
“The next step will be exploring the possibilities of concentrating different enzymes together, which is highly promising in advancing their catalytic ability, in creating new chemicals, as well as in processing industrial waste, in an efficient fashion,” Olvera de la Cruz said.
Posted: Feb 02, 2018
Kirigami Nanofluidic Devices
(Nanowerk Spotlight) Recent research in nanofluidics has adopted reconstructed layered two-dimensional (2D) sheets (such as graphene oxide or clay) as a promising material platform for nanofluidics. These membranes contain a high volume fraction of interconnected 2D nanochannels.
This nanochannel fabrication method is straightforward and scalable, and does not rely on lithography or etching.
"Compared to other scalable nanofluidic materials such as anodized aluminum oxide membrane, block copolymer membrane and nanofluidic crystals, a unique feature of such layered membranes is that the channels are horizontally aligned and the channel height (i.e., interlayer spacing), which is responsible for confinement of the electrolyte, remains uniform throughout the entire thin film," Jiaxing Huang, a Professor of Materials Science and Engineering at Northwestern University, tells Nanowerk. "Therefore, nanofluidic ionic transport properties are maintained regardless of the lateral dimensions or shape of the 2D nanochannel network."
The researchers termed this process, which opens up a range of new opportunities for manipulating ionic transport by tailoring the shape of the films, kirigami nanofluidics. They reported their findings in Materials Chemistry Frontiers ("Kirigami nanofluidics").
The work shows that these 2D membranes behave like 'ionic wafers', which can be patterned by kirigami to realize device functions such as diodes.
"Although these ionic functions are still quite simple, compared to electronic devices, they did show that ionic transport through a layered membrane, which is a nanoscale phenomenon, could be tuned by tailor cutting the macroscopic shape of the film," notes Jun Gao, the paper's first author. "We hope the mechanistic understanding and the demonstrations in this work can help people better understand ionic transport through these 2D films, which is useful for designing large scale nanofluidic channels arrays for separation purposes."
Compared to the classical ionic diodes, which typically are made of channels with asymmetric ends such as a conical nanopore (Figure 1a), the 2D nanochannels have uniform channel size (i.e. interlayer spacing) throughout the entire volume of the film (Figure 1b). This simplifies the factors affecting ionic transport, making it possible to control the overall ionic conductance by tailoring the ionic resistance at the channel-reservoir interfaces.
For example, a graphene oxide film with asymmetric ends, such as a T-shape, exhibits ionic rectification (Figure 2d). While shapes with symmetric ends, such as a diode-sign, exhibits no rectification (Figure 2c).
Kirigami nanofluidics allows the fabrication of nanofluidic devices and the control of their performance in a very straightforward way. For example, the ionic current rectification ratio can be adjusted by 'tailor cutting' the geometry of the film, which has been quite difficult in previous works.
Nanofluidic devices are ionic analogs to semiconductor devices. Kirigami-made resistors and diodes can be connected to realize some simple logic functions such as the AND gate and OR gate.
"An electrolyte confined in nanochannels exhibits unusual behaviors owing to strong influences from the channel walls," explains Erik Luijten, a Professor of Materials Science and Engineering at Northwestern University. "When the size of a charged channel is comparable to the Debye length of the electrolyte, co-ions are effectively repelled from the channel and counterions are concentrated, leading to the so called unipolar ionic transport properties with the counterions as the majority charge carrier."
The team first hypothesized that such nanochannels can be readily formed by stacking up 2D sheets, such as graphene oxide. Indeed, they had previously demonstrated that films of graphene oxide or clay sheets contain massive arrays of parallel 2D nanofluidic channels.
In this regard, films of 2D sheets are conceptually analogous to extrinsically doped semiconductors, a material foundation that has enabled the realization of numerous electronic devices and integrated circuits.
In the current work, Huang's team showed that like semiconductor wafers, papers of graphene oxide can also be 'patterned' to realize some analogous functions such as ionic diodes or logic circuits. The patterning is done by cutting the film into various shapes.
In one demonstration, Huang's team showed that programmable rectification ratio can be realized in multi-armed films, by varying the numbers of arms for the source and drain, respectively.
Going forward, the scientists are interested in creating nanofluidic devices with active gating function, which would allow us to manipulate nanofluidic transport with more freedom.
Monica Olvera de la Cruz, a co-author of the paper and a Professor of Materials Science and Engineering at Northwestern University, concludes that "It will be very exciting to realize some form of gating mechanism to make ionic transistors, and to integrate all of these device elements to achieve a bit more complex logic or even computing functions."
By Michael Berger – Michael is author of two books by the Royal Society of Chemistry: Nano-Society: Pushing the Boundaries of Technology and Nanotechnology: The Future is Tiny. Copyright © Nanowerk
Honors and Awards
Monica Olvera de la Cruz and Chad Mirkin are recognized by Sherman Fairchild Foundation
Northwestern University’s Monica Olvera de la Cruz and Chad A. Mirkin have received significant five-year grants from the Sherman Fairchild Foundation in support of their innovative materials science research.
Olvera de la Cruz, the Lawyer Taylor Professor of Materials Science and Engineering in the McCormick
School of Engineering, will receive $4.5 million to accelerate the discovery of new functions and application of synthetic structures, including hybrid biological and synthetic assemblies. She will focus on structures that mimic and enhance the action of protein membranes and cells to better understand biological functions, with applications in environmental remediation and health care. The project promises to push forward the field of biomimicry, which seeks to solve pressing problems by emulating nature.
Olvera de la Cruz is recognized internationally for her contributions to analyzing, modeling and designing new materials that mimic effective biological processes. She also is a professor of chemical and biological engineering, chemistry, and physics and astronomy and director of the Center for Computation and Theory of Soft Materials.
Mirkin, the George B. Rathmann Professor of Chemistry at the Weinberg College
of Arts and Scienc es, will receive $5 million to develop a novel design method called “nanocombinatorics.” The chemistry-based approach would systematically and comprehensively screen innumerable nanomaterial combinations and identify the most promising for a given area of study or application. The resulting encyclopedic resource of high-performance nanomaterials could be used in energy production, environmental remediation, health care, computing technology and homeland security.
Mirkin is world-renowned for his nanoscience expertise and invention of spherical nucleic acids and the development of biological and chemical diagnostic and therapeutic systems based upon them. He also is director of Northwestern’s International Institute for Nanotechnology and a professor of medicine, chemical and biological engineering, biomedical engineering, and materials science and engineering.
The funds raised through We Will. The Campaign for Northwestern are helping realize the transformational vision set forth in Northwestern’s strategic plan and solidify the University’s position among the world’s leading research universities.
Breaking the Protein-DNA Bond
Study finds that free-floating proteins break up protein-DNA bonds at the single-binding site
Apr 4, 2017 | Amanda Morris
The verdict is in: too many single, flirty proteins can break up a strong relationship.
A new interdisciplinary Northwestern University study reports that the important protein-DNA bond can be broken by unbound proteins floating around in the cell. This discovery sheds light on how molecules self-organize and how gene expression is dynamically controlled.
“The way proteins interact with DNA determines the biological activity of all living organisms,” said John F. Marko, professor of molecular biosciences, physics, and astronomy in Northwestern’s Weinberg College of Arts and Sciences. “Inevitably, any malfunction in this interaction network can lead to malevolent conditions. It is paramount to precisely understand the interaction mechanisms underlying protein-DNA associations.”
To understand this vital relationship, Marko led a study examining the sites where a single protein binds to DNA. Strands of DNA have specific sites on which other molecules can bind and become a part of the DNA’s genetic code. One type of DNA-binding proteins, called transcription factors (TF), are key players in the transcription of genetic information from DNA to messenger RNA (mRNA) to produce new proteins or other types of RNA. TF proteins control the biological processes in living cells by binding and unbinding to DNA. In the experiment, Marko and his team developed a concentration of TF proteins bound to DNA mixed with unbound TF proteins, which competed with the bound proteins for their binding sites. They observed that unbound proteins caused the bound proteins to dissociate from the DNA. The unbound proteins then stole the newly available single-binding sites.
“Our experiments show that dissociation happens on the level of a single protein-DNA interaction,” Marko said. “This is new information for the field.”
Supported by the National Institutes of Health and National Science Foundation, the research was published online this week in the journal Proceedings of the National Academy of Sciences. Ramsey Kamara, a former postdoctoral researcher in Marko’s laboratory, served as the paper’s first author. Northwestern Engineering’s Monica Olvera de la Cruz, the Lawyer Taylor Professor of Materials Science and Engineering, coauthored the paper.
Olvera de la Cruz led the development of a theoretical model and performed molecular dynamics simulations to show the prevalence of the protein-DNA break up at the single-binding site due to the competitor proteins. This disproves former beliefs that protein-DNA bonds were unaffected by unbound proteins and instead resulted from more “cooperative” interactions among many molecules, large protein clusters, or long DNA segments.
“Our results suggest that protein-DNA dissociation could have a profound effect on the dynamics of biological processes that depend on protein binding in vivo,” Olvera de la Cruz said. “This may be an important factor to take into account when modeling gene expression in living cells.”
Olvera de la Cruz to Receive 2017 APS Polymer Physics Prize
The prestigious award honors her contributions to the theoretical understanding of polymers
Oct 17, 2016 | Amanda Morris
Northwestern Engineering’s Monica Olvera de la Cruz to receive the 2017 Polymer Physics Prize from the American Physical Society (APS).
Olvera de la Cruz was selected for her “outstanding contributions to the theoretical understanding of polymers and the effects of electrostatic interactions on their structure and properties.” She will officially accept the award at the APS March Meeting next year.
“I am honored to receive the 2017 APS Polymer Physics Prize,” said Olvera de la Cruz, the Lawyer Taylor Professor of Materials Science and Engineering in Northwestern’s McCormick School of Engineering. “It is given to highly distinguished scientists, including three Nobel Prize winners — Pierre Gilles de Gennes, Peter J.W. Debye, and Paul Flory — and my PhD adviser Sir Sam Edwards.”
Olvera de la Cruz’s research focuses on the development of models to describe the self-assembly of heterogeneous molecules, including amphiphiles, copolymers, and synthetic and biological polyelectrolytes. She also studies the segregation and interface adsorption in multicomponent complex fluids. Her group’s work has resulted in a revised model of ionic-driven assembly, and its investigations into soft and condensed matter physics have advanced scientific knowledge and opened new research fields of technological importance.
“Polymers have fascinating physical properties,” Olvera de la Cruz said. “They have attracted studies by scientists and mathematicians for decades, given that they are one-dimensional molecules with nanoscale dimensions that are capable of taking multiple conformations.”
A member of the National Academy of Sciences, Olvera de la Cruz has received several other honors and awards, including the Engineering and Applied Sciences Cozzarelli Prize from the National Academies, a National Security Science and Engineering Faculty Fellowship, an Alfred P. Sloan Fellowship, and the NSF Presidential Young Investigator Award and the David and Lucile Packard Fellowship in Science and Engineering. She is also a fellow of the American Academy of Arts and Sciences and the American Physical Society.
Honors and Awards
Chad Mirkin Honored at Awards Ceremony in Israel
News Link
Northwestern’s Chad Mirkin (center) accepts the 2016 Dan David Prize in the Future Time Dimension from Joseph Klafter (left), president of Tel Aviv University and chairman of the Dan David Prize Board of Directors. Northwestern President Morton Schapiro (right), along with other Northwestern officials, also attended the May 22 awards ceremony. Photo by Hadari Photography